About this essay. I wrote it to be self-explanatory enough on its own. Still, my other essays might help you get a better feel of some terms I use here, like “differences”, “alignment”, and “trees of possibilities”. Links to the relevant essays are in the text.
Out of the many charming short stories in David Eagleman’s Sum: Forty Tales from the Afterlife, one in particular has stuck with me. Its title is Ineffable and it’s short enough to be read in five minutes. This version of the afterlife (each story in the book gives an alternative variant) is not for people, but for the larger things that their associations create: platoons live during the war and die when their soldiers disband; stage plays have a short life of their own, beyond that of individual actors; stores and congresses perish long before the humans that made them possible. All of these ephemeral entities, the narrator tells us, go on to exist forever in their own special heaven.
Although it is difficult for us to imagine how these beings interact, they enjoy a delicious afterlife together, exchanging stories of their adventures. They laugh about good times and often, just like humans, lament the brevity of life. The people who constituted them are not included in their stories. In truth, they have as little understanding of you as you have of them; they generally have no idea you existed.
I find this to be a wonderful thought. I don’t believe in souls or in life after death, but if they did exist for people, why shouldn’t they exist for projects, for companies, or societies? Aren’t they alive in some sense, don’t they have their own stories, personalities, and vices above and beyond those of the people within them?
This is a deep rabbit hole to jump into. When you think about it, so many words in our daily vocabulary refer to kind-of-abstract-but-real entities like that. Many of these notions involve living things, like “school”, “herd”, and “ecosystem”, but we also have tons that are not made of life forms: “river”, “cloud”, “galaxy”, and so on. Intuitively, it feels like all these words point to more than mere groups of things like human beings and vapor droplets. They are more than the sum of their constituents. They seem to have some level of independence from their members. But what are they exactly?
It turns out that science has a word for this concept, and that it’s a hot topic. They’re called emergent phenomena, and the general word for their appearance is emergence. It’s a crucial idea in modern science. Its proponents hail it as the best paradigm to understand the world. Its detractors might quibble about what it is exactly, or how important it is, but very few outright deny that it is a thing.
Emergence isn’t really a new idea. It happens all around us, and for the most part it isn’t that interesting. It seems like it’s so common that scientists, well… they “forgot” to study it for a few hundred years! From Descartes and Newton up to the late 19th century, everyone was having a lot of success taking things apart, analyzing what stuff was made up of, and no one was doing research on how new properties emerge when you put things together.
But we’ve come to realize that emergence also does some very interesting things, and it’s finally been accepted as something worth studying more in depth. The problem is, it can be confusing.
Triviality on the Grills
Like some other scientific terms—“information” and “entropy” come to mind—, “emergence” suffers from a stubborn aura of mystery, a smoky intangibility, an almost magical nuance. It has something to do with holism, so it must be pseudo-scientific new-age fluff, right?
It doesn’t help that when you open the Wikipedia page for emergence, or watch an online lecture about it, you’re immediately thrown into philosophical disquisitions of “weak” and “strong” emergence, into its battle against “reductionism”, and similar intellectual roller coaster rides. In other words, you find a barrage of abstract philosophical terms and debates that might be interesting for the specialists, but probably aren’t what the majority of non-philosophers need to care about. Most of us want to know what emergence is in practice and how we can use this idea to fare better in our lives. Explaining things like “epistemological” vs “ontological” emergence is about as useful for the layperson as expounding on the nature of the Self or the meaning of causation. An intriguing thought for some, a distraction for most.
To top it all, scientists in different fields use the word “emergence” is subtly different ways. Which is the right one in general? One more obstacle to overcome.
At the end of the day, emergence isn’t a difficult idea at all. To put it like network scientist Petter Holme, emergence is profoundly trivial—profound, because it’s about the very structure of reality, and trivial, because we all see and work with it just fine every day. We all understand emergence already. We just struggle to see how far-reaching it is.
The trick to grok it is to go by degrees, from simple to complex.
Example 1: Tongs
For starters, imagine a pair of grill tongs, like the ones they use to flip food at a barbeque.
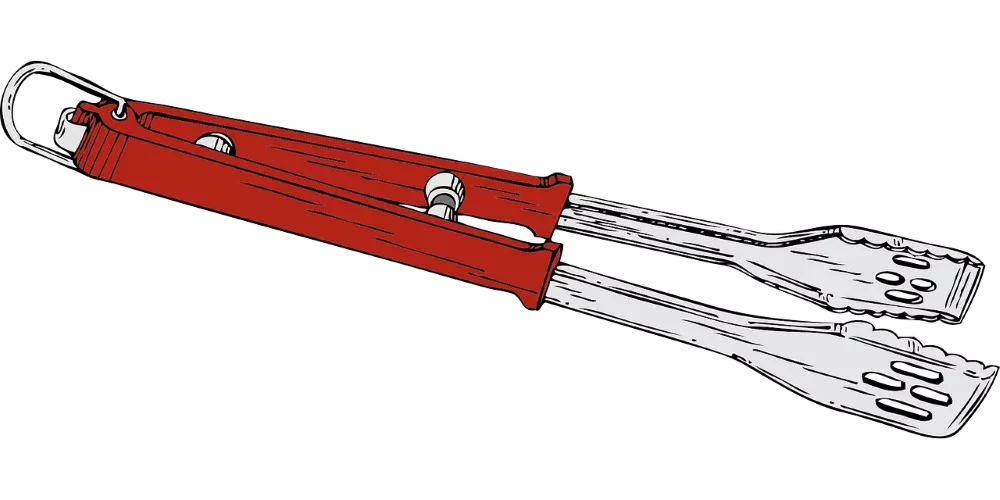
In simplified form, this “tongs system” is made of two long metal strips connected by a spring or elastic joint or two. When you squeeze it around a juicy morsel, its tree of possibilities may look similar to this:
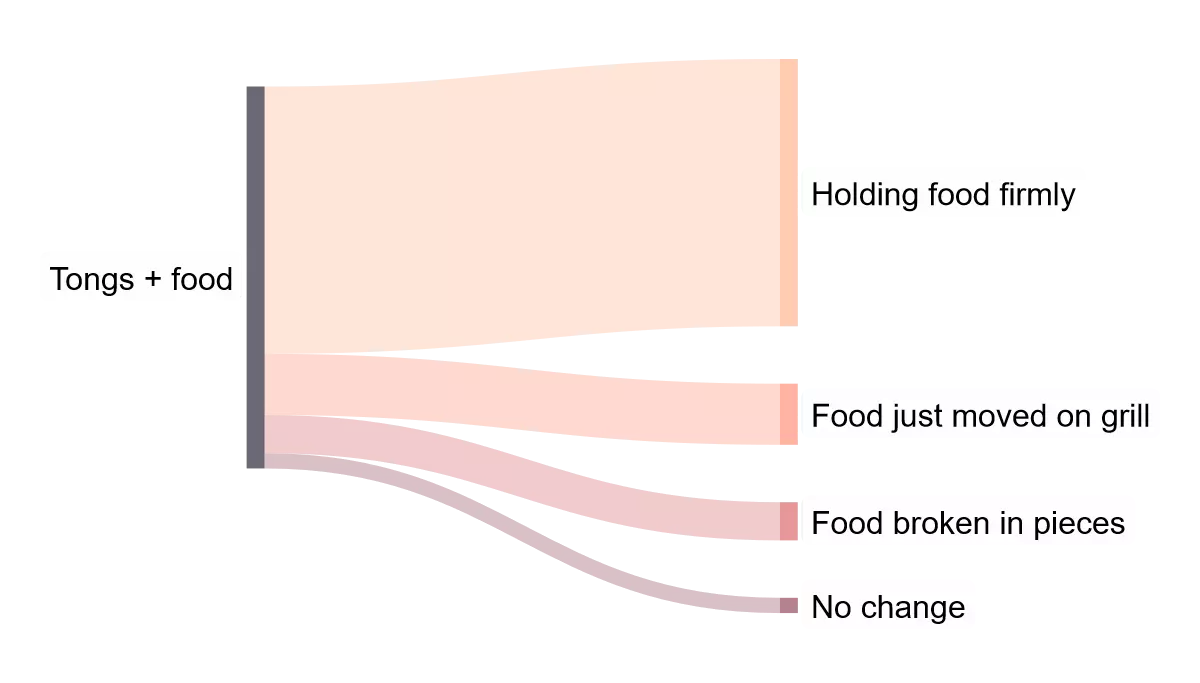
It’s very likely that you will succeed at gripping the piece of food, but sometimes you might fail to pick it up, or grip it tight enough to break it down in two or more pieces. In some rare cases you may be so clumsy that you fail to even nudge the item at all. (Exactly how thick each “branch” of the tree above should be drawn depends on a few things, not least your “barbecuing chops”. But the thickness isn’t important in this discussion.)
Next, unhinge the poor instrument, snap one of its two arms off, and use that single arm (“tong”?) to handle the food again.
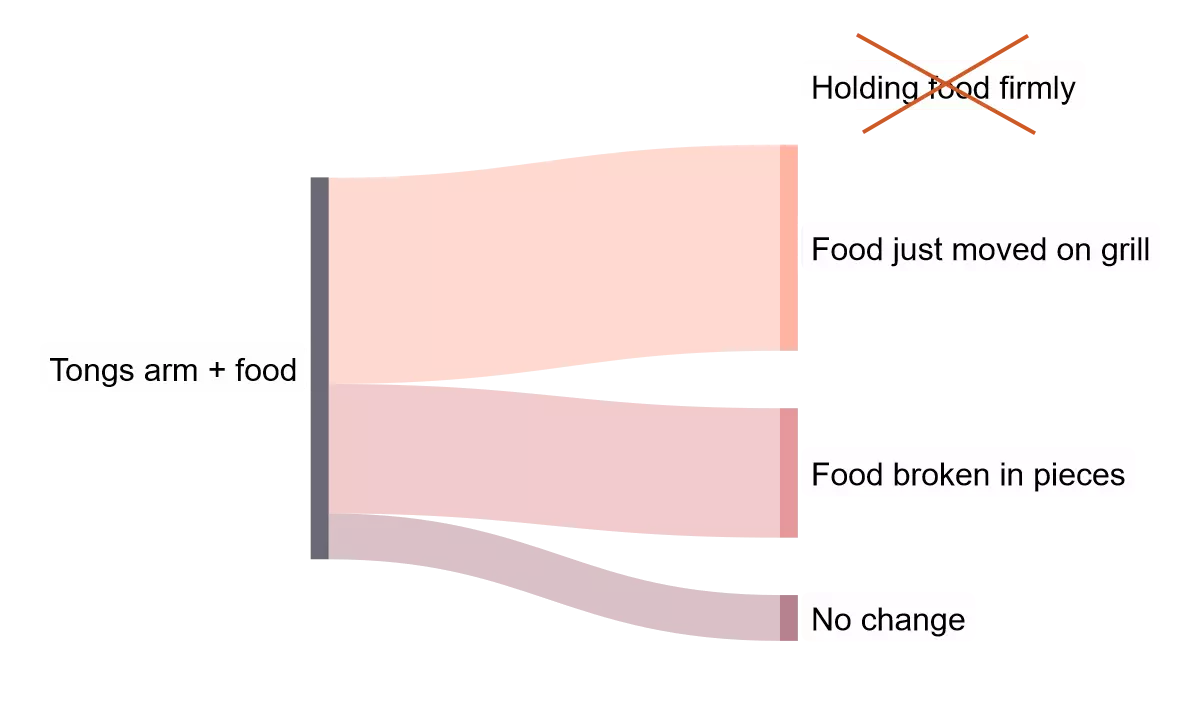
Some of the results you could get with the full tongs are still possible with only one of the arms. You can toss the foodstuff around, you can hack at it to break it apart, maybe you can even manage to flip slices (careful not to drop it through the bars!). But there is at least one thing you definitely can’t do with it now: holding the food firmly.
This is emergence at its most basic. With the intact tongs you can do something different from what you could do with a part of the tongs. It’s not that tongs with two arms can do “twice as much” of what a single arm can do. Even with the exact same materials and parts, changing the structure of the system—the alignment of its parts—makes a qualitative difference.
If all this feels obvious to you, it’s because it is. There is no hidden magic. Sure, I could climb on a chair and yell, “Behold! Upon assembling two insipid components, a whole new branch of the possibility tree hath materialized from the very ether!” and it might sound a little mysterious, but honestly, it’s a stretch. Emergence, in this sense, is trivial. We see it all the time.
The same argument holds for the head and handle of a hammer, for the parts of a wheel, and for almost anything that needs to be built in a given way to work as intended. We are master aligners of stuff. We make new possibilities emerge all the time. It’s how we humans destroy control the world.
An Emergence Staircase
Instead of jumping straight to the most interesting cases of emergence—which can be jarring and mystifying—let’s ramp up our intuition with a series of examples of increasing complexity. Each example includes a depiction of its tree of possibilities.
(As always with these diagrams, we should keep in mind that these are all simplifications, that they can only be drawn when we have a purpose in mind, and that the exact branches and their thicknesses depend on how much we know about those systems. In fact, I’ll give all branches the same width in these examples, to keep the spotlight only on which branches exist. Quality, not quantity.)
Plain Complementarity
The simplest kind of emergence is what we’ve just seen above, but it doesn’t hurt to have more examples. We might call this emergence plain complementarity, or feature emergence. It’s about a few smaller systems each contributing in their own special way to the new emergent effect.
Example 2: Heavier-Than-Air Flight
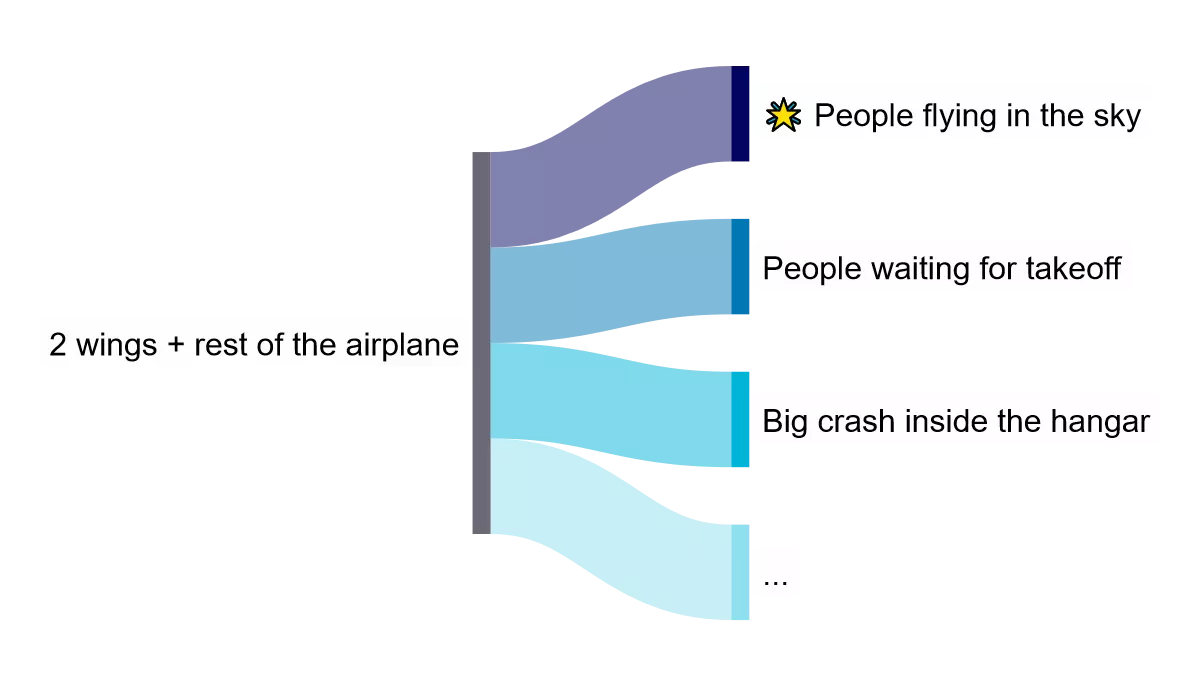
Remove a wing from the airplane, and you won’t make it to your trip destination. Only with both wings (and a lot of other parts) in the right places will the emergent marvel of flight allow you to enjoy those tropical beaches.
Example 3: Two-Body Orbits
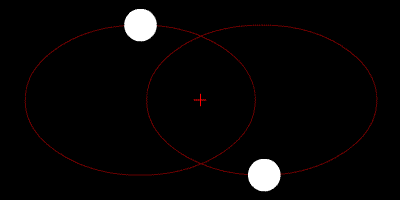
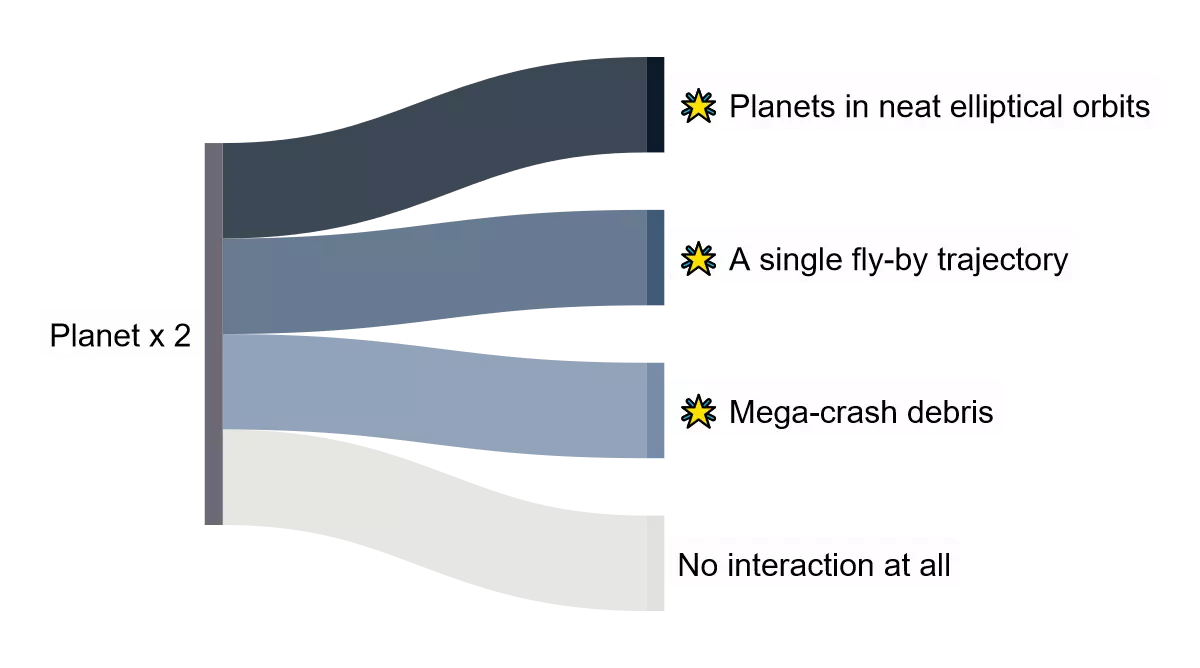
A single planet in the middle of empty space will move in a boring straight line. Introduce a second planet (or moon, or star) nearby, and the reciprocal gravitational pull will make them move in very predictable ways: neat ellipses described by Kepler’s laws. Exactly what will happen depends on the alignment of the two bodies: their initial positions, masses, and speeds.
Advanced Complementarity
As you add more elements, and as the number and types of interactions between them increase, the results get more interesting and sometimes outright puzzling. But they are essentially the same kind of emergence as the previous examples, so we can call them “advanced complementarity”.
Example 4: Cooking
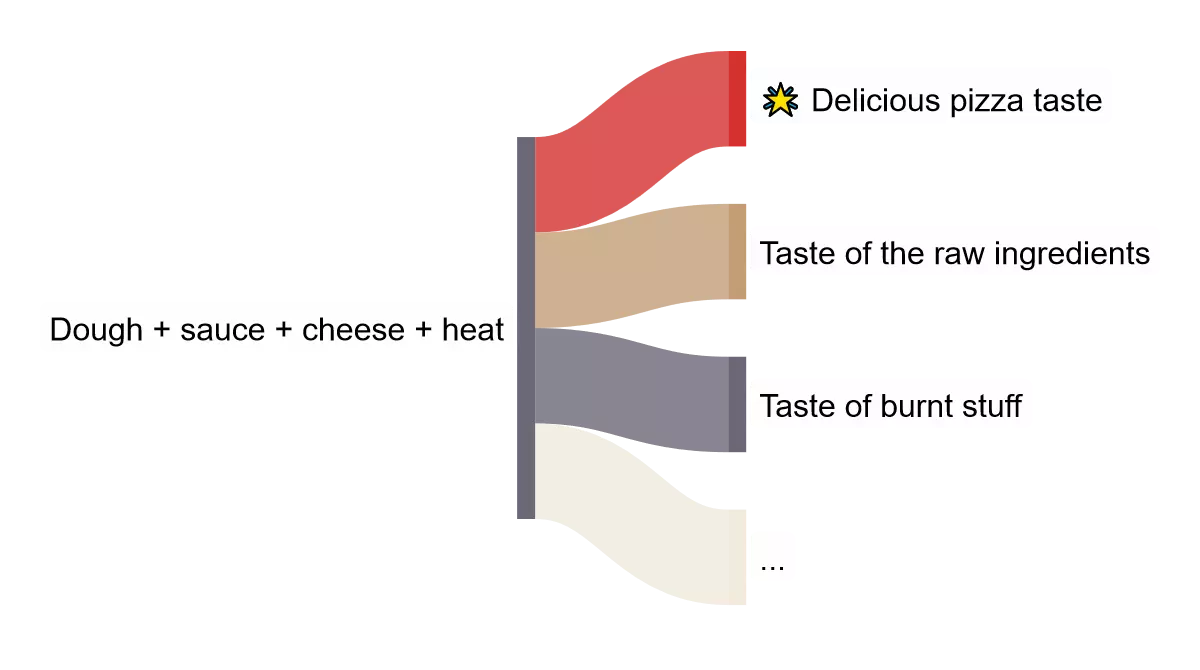
Eating a hot pizza right out of the oven is not the same thing as eating each of the ingredients separately. Mixing liquid cheese into the dough itself isn’t going to work either. You need the right elements combined and processed in the right order to produce its unique flavor. Compared to the tongs and airplane examples, it is harder to understand exactly how the different materials complement each other. The interactions are happening at the tiny scales of molecules and cells. But they’re still cases of feature emergence.
Example 5: Three-Body Orbits
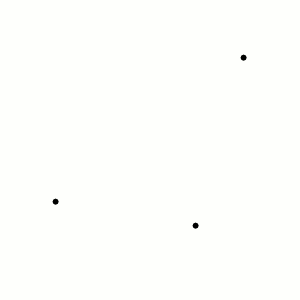
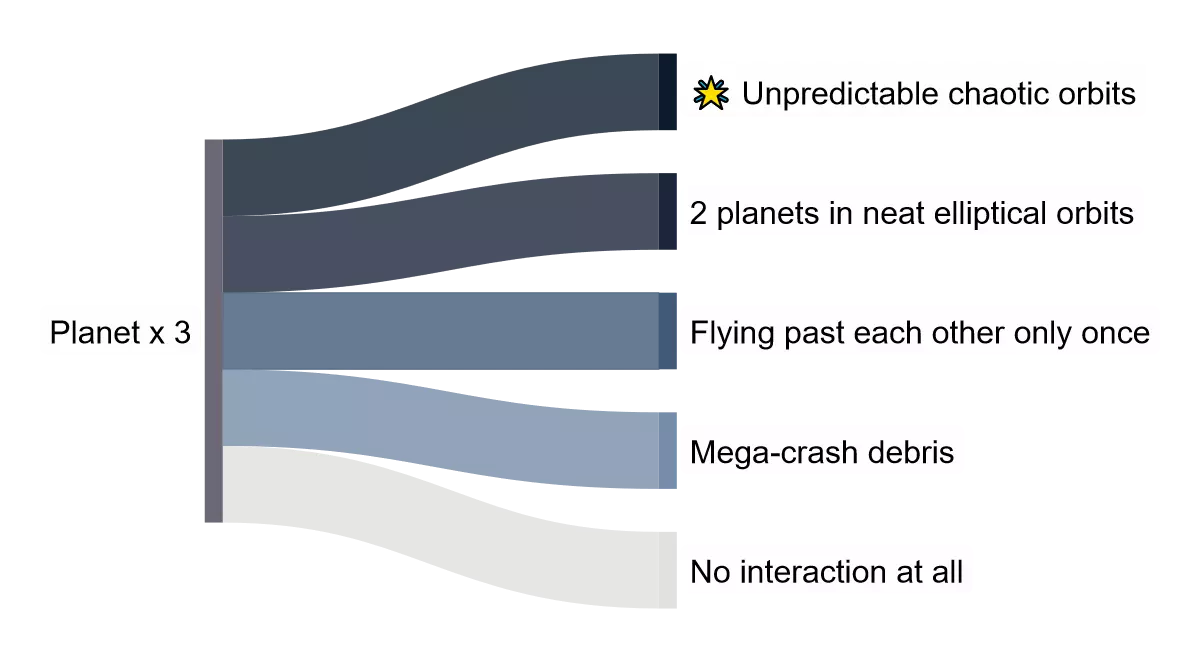
Return to Example 3 and add a third planet of similar size. You might expect to have more or less the same kind of motion, like the usual elliptical orbits, but with three bodies instead of two. Not in general!
As long as the planets are all close enough to each other, what you get is a whole world of absolutely insane orbits that defy even the wildest nightmares of Johannes Kepler. This is the famous Three-Body Problem, a long-standing mathematical problem known to be unsolvable (unpredictable) in general. Two bodies lead to periodic elliptical orbits; three bodies lead to chaos.
Example 6: Protons
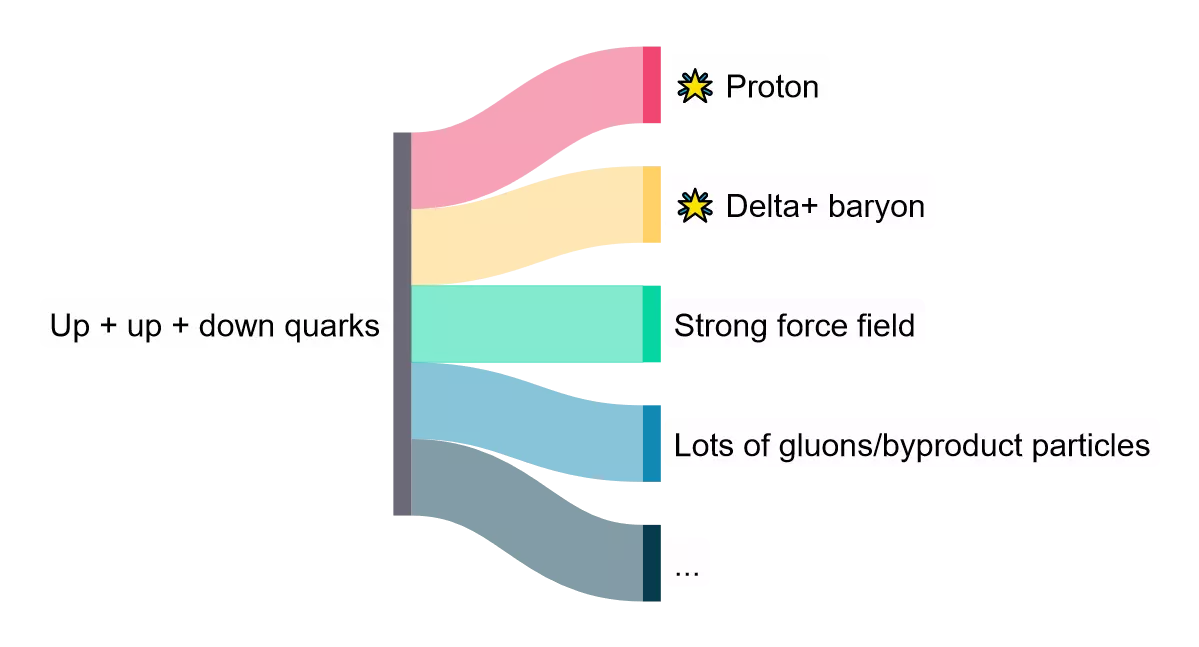
We say that protons are made of two up quarks and one down quark, but another way to put it is that when those quarks get together something with the mass and charge and other properties that we call “proton” can be said to exist. Aligned differently, the same quarks produce a particle with very different properties, called a delta+ baryon. A different combination of the same quarks (up + down + down), will lead to yet another particle, which we call “neutron”.
What’s more striking is that the mass of the proton is not the sum of the masses of the three quarks, and it does things very different from what individual quarks can do, like teaming up with other protons and neutrons to form atomic nuclei. In other words, protons (and many other composite particles) are emergent phenomena. (We don’t understand quantum physics enough to tell if that’s the case for all particles, but that’s a story for another day.)
Example 7: Software
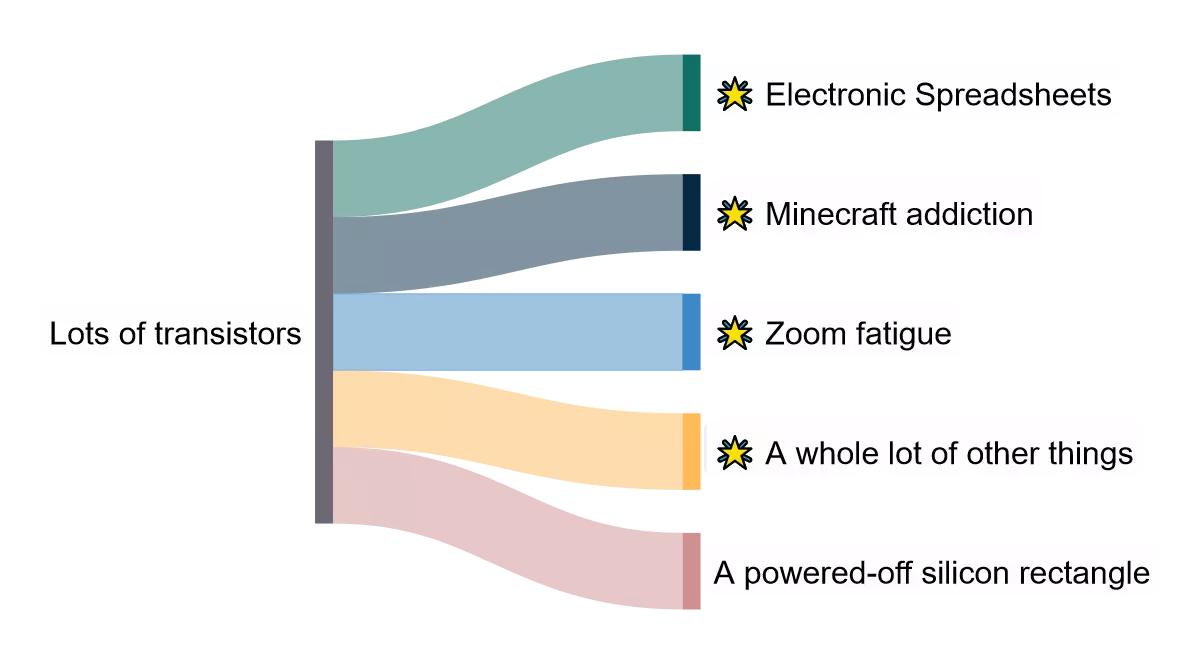
At the core of every computer is a Central Processing Unit (CPU), a.k.a. its processor. A modern CPU is essentially a grid of billions of transistors (and other things like wires and resistors, but the transistors are the most interesting bit).
Each of those transistors is a simple electronic on/off switch. It’s almost as boring as the switch on your bedside lamp. But when arranged and connected in specific ways, they allow us to compute basically anything we can imagine. Software is the emergent behavior of the hardware, and so are the effects of software on our lives.
While this example might also belong to the next category of emergence I count it as a case of advanced complementarity because the transistors and other circuits are grouped into regions with complementary goals: control units, caches, clocks, etc. Remove one of those regions, and the software won’t emerge.
Context Emergence
The next “level” of emergence isn’t really a level. It’s not so much a question of the number of elements any more—although the number often matters—nor about parts doing complementary jobs. In these cases, whole new laws and behaviors emerge out of subsystems that might all be doing more or less the same thing!
I like to call this last category context emergence, because they are all groups of simpler systems combining in ways that turn them into a “context”, a unique kind of space in which strange new things can happen. Each of those structures becomes a canvas onto which specific physical laws and, often, whole new worlds of existence draw themselves. Another apt name would be physics emergence, as opposed to mere feature emergence.
Example 8: Waves On Water
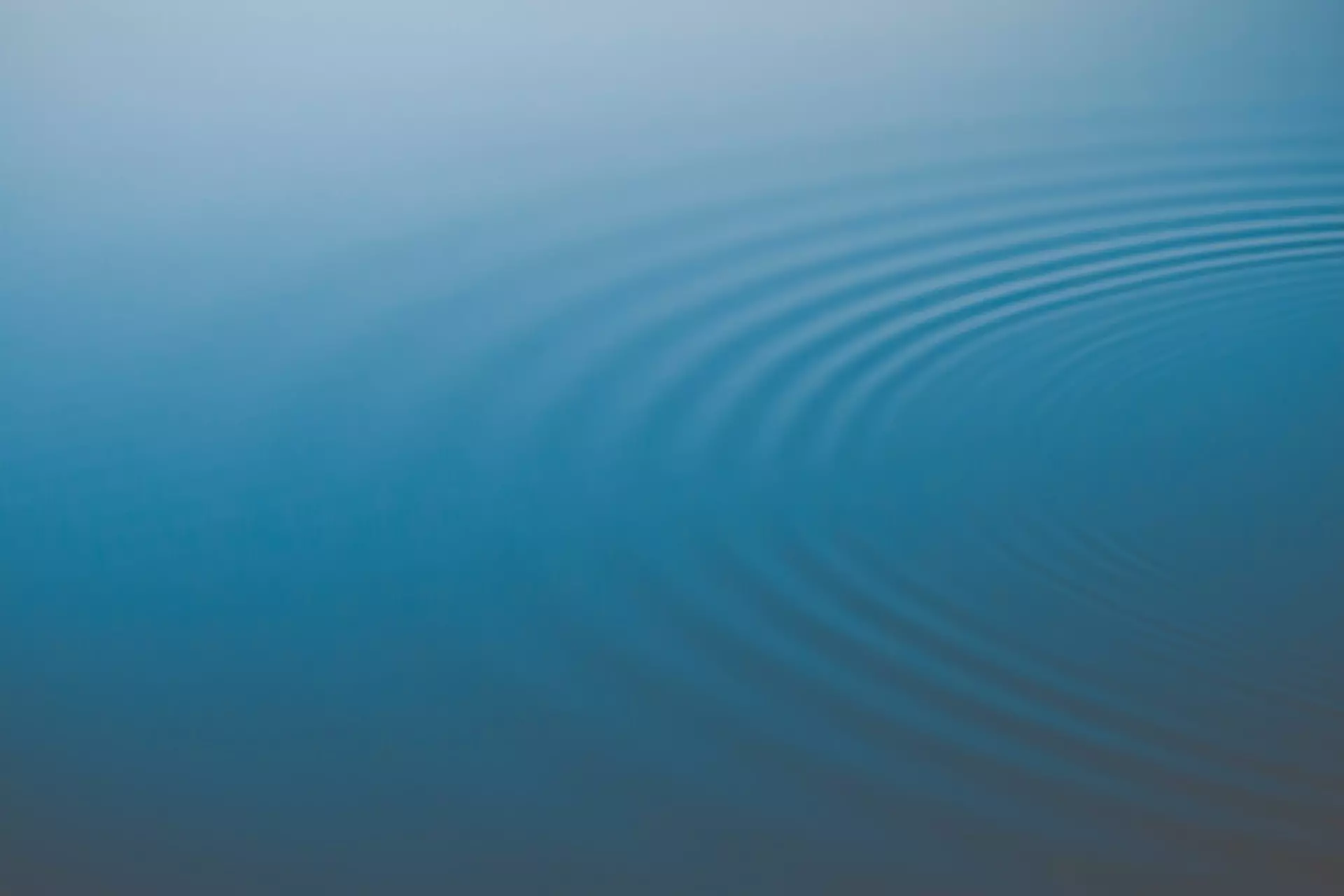
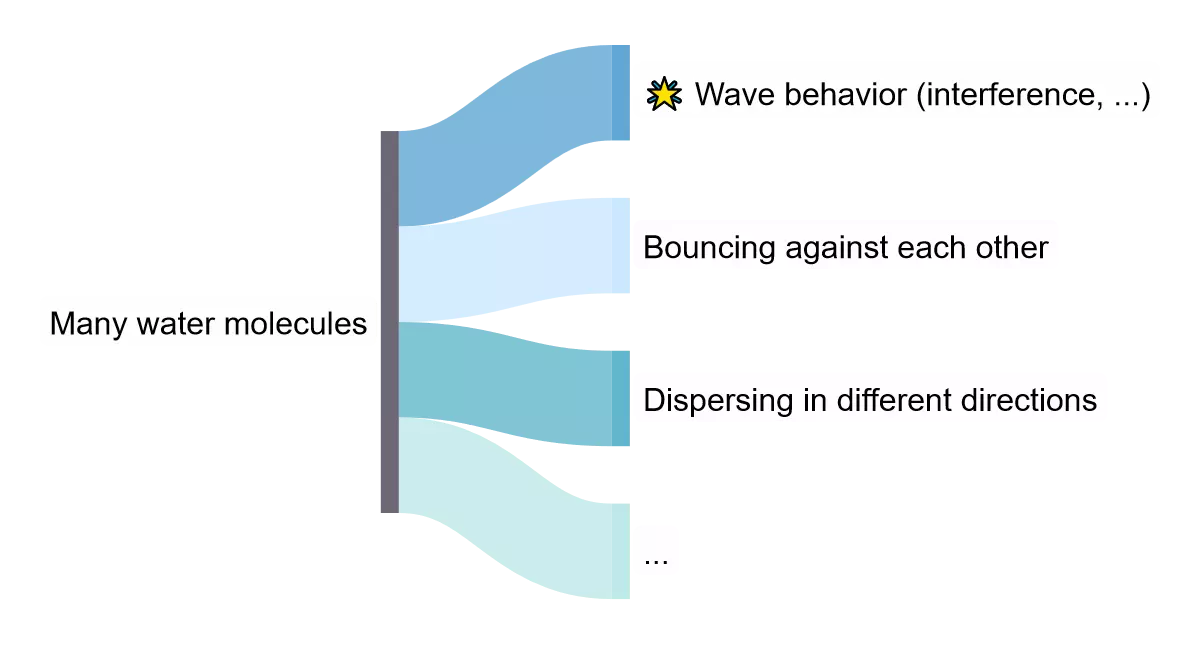
Put enough water molecules together and a new kind of synchronized motion becomes possible, which we call waves. But the oscillation of water isn’t everything that emerges out of the blue: these waves follow a set of laws of their own. Waves propagate with specific speeds, shapes, and wavelengths. When two waves overlap, they make “interference” patterns. When they hit an obstacle, they rebound in what physicists call “diffraction”.
All of these emergent laws can be written mathematically (for example, the characteristic wave speed in shallow water is simply meters per second) and they don’t require any knowledge of the individual water molecules that are doing the actual shaking. An H2O molecule on its own can’t be diffracted. It doesn’t have a “characteristic speed” depending on how far it is from the bottom of the tank it’s in. Those notions only begin to make sense when a lot of them are aligned in a special way that we call “liquid water”. Only then do they become a context where clear wave equations apply.
Waves, of course, happen in many other things that are not water, like air, ropes, solid objects and even crowds (you need at least three people to make a human wave). The exact laws vary a little from context to context, but they all resemble each other.
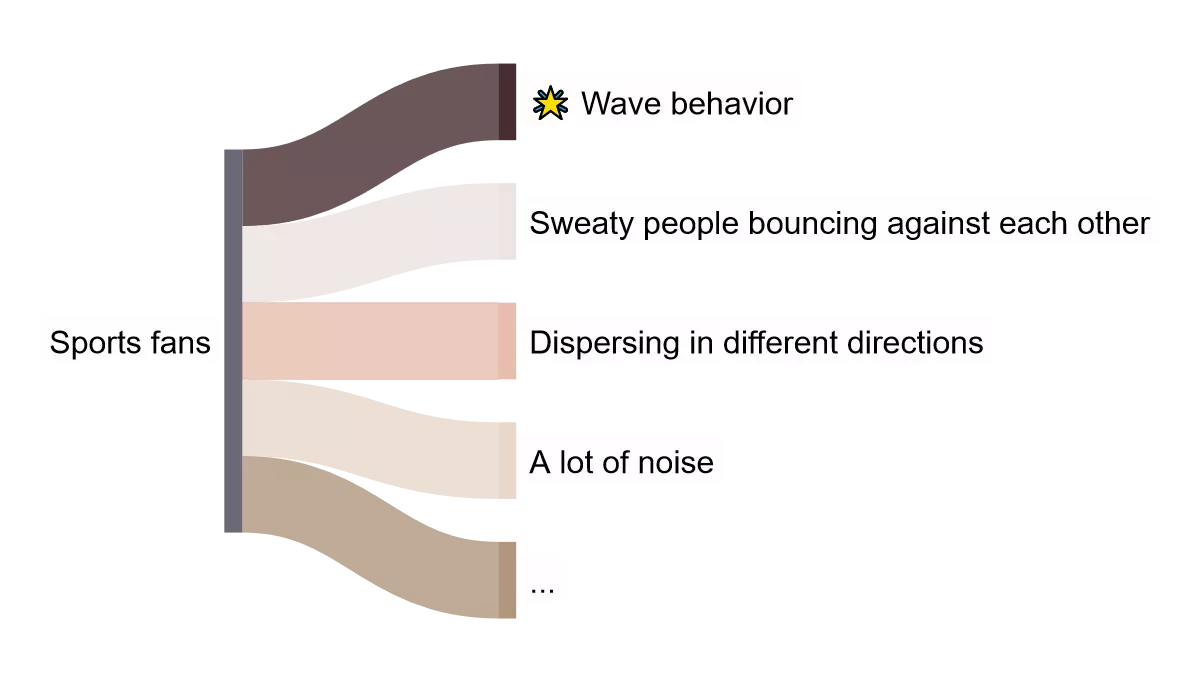
Example 9: Swarms
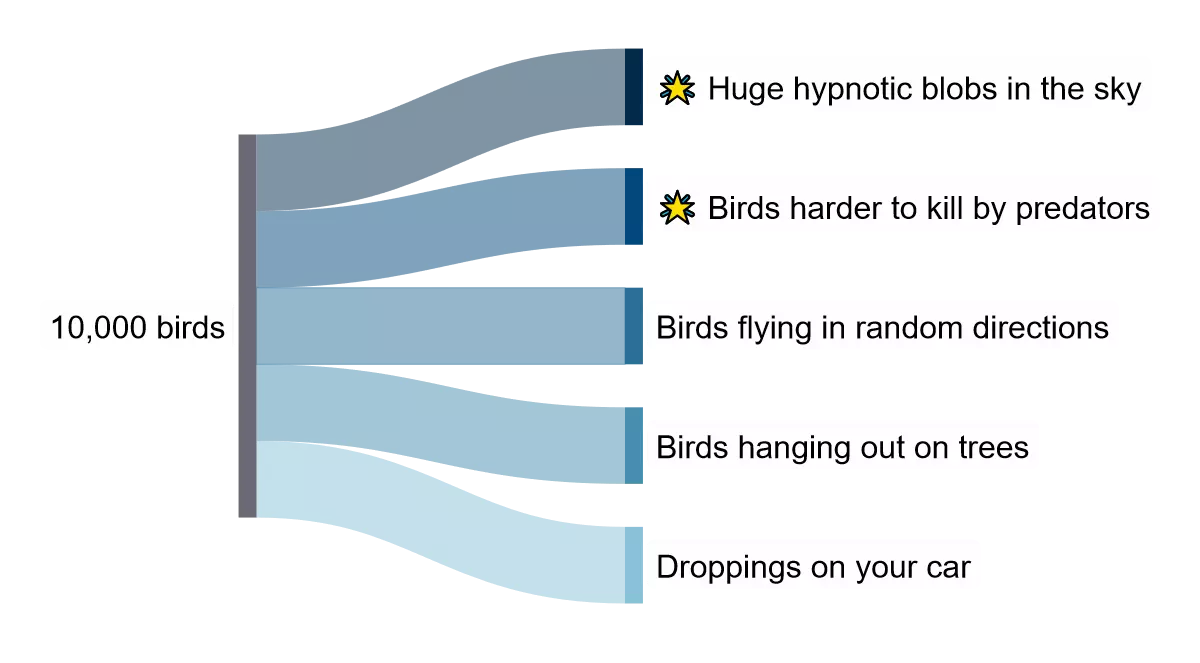
Each bird (or fish, or sheep) is looking at its peers and reacting as its simple instincts tell it to. There is no leader or controller guiding the group to make certain shapes. The mesmerizing motion emerges from their interactions, and it has its own tendencies and laws which we can study mathematically).
More Examples
Most things with this kind of emergence are so complex that I have no hope of drawing meaningful trees of possibilities for them. They include things like:
- Thermodynamics: behaviors of matter like temperature and pressure—and the laws they follow—emerge from their simple molecular building blocks.
- Chemistry: the relatively simple physics of molecules leads to all sorts of crazy chemical reactions and endless ways in which different materials interact with each other.
- Solid state physics: when a lot of molecules condense, they take on a whole new set of properties that makes everything solid around us possible.
- Life: a bunch of molecules, mostly carbon-based, aligned in patterns that make them move, regenerate, and replicate those same patterns.
- Sociology: social classes, progress, religions, wars and other baffling things like that all emerge from billions of small interactions between human beings. No one is in control (and not for lack of trying).
- Consciousness: the classic example of ultimate emergence. How can a pile of dumb nerve cells lead to sophisticated conscious thought and sensations?
- And a billion more things. These contexts also overlap, build on top of each other, and disturb each other in all sorts of ways.
This catalog is impressive, but I hope it doesn’t feel magical. I can’t stress this enough: the juice is in how things are organized. Nothing is popping out magically out of thin air. Like for the case of the tongs, new things are possible when new ways to align and interact are put in place.
Still, context emergence may be non-magical, but it is by far the most studied, yet least-understood, kind of emergence. It’s so important that some scientists (especially physicists) typically use the word “emergence”, without any other qualifier, to mean what I call “context emergence”, ignoring the simpler complementarity categories altogether—a big source of confusion, if you ask me.
The Tough Bits
Emergence is at play when the behavior of a system only makes sense by zooming out and looking at the bigger system it’s in. You can’t explain how a flat piece of steel can keep food suspended near it unless you zoom out and look at the whole “tongs system” that includes it. And training your binoculars on a single bird will make it look utterly insane unless you observe the flock swarming around it.
Another way to put it is that a difference (property, behavior, law) is emergent if it can only happen when all the different parts (complementarity) or enough of the same parts (context emergence) are present and aligned in a specific way.
You don’t need a PhD or even high-school education to understand that definition. Why, then, do people treat it as a difficult, slippery topic, shrouded in an aura of mystery? I think it is because, even though the idea is simple, in many cases it turns out to be almost impossible to model, predict, and control. It’s a bit like a game of Jenga in ultra-hard mode: easy rules, hard game.
There are three major reasons why emergence is hard to predict.
Combinatorial explosions are the first problem. For each part you add to the whole, there is a rapidly growing number of options to choose from: the kind of parts to add, the order in which they’re added, the way they align. The number of possible changes goes astronomical very soon, so that it’s impossible to try them all. There will always be mountain-loads of structures which might lead to some kind of emergence, but you’ll die long before you get the chance to try them—even if you had a supercomputer to simulate them.
The second reason emergence is tough to crack is that many emergent effects are what’s called nonlinear behaviors. Nonlinearity means that if you doubled the inputs to the system, in general you’ll get something other than just “double the effects”. An example might help here.
Imagine a small island populated by rabbits and rabbit-eating foxes. After a while, the number of rabbits and foxes, and the amount of rabbit-food, reaches an equilibrium: an ecosystem has emerged.
Now, let’s say that you really like rabbits, and want to see more of them on the island. At first, you bring a few rabbits from elsewhere, and the effect is that the rabbit population simply grows by that much. That looks linear and predictable enough! But as you keep adding rabbits, a few at a time, they’ll slowly deplete the food on the island, until suddenly they’ll run out of things to eat and begin dying of starvation. At the same time the foxes will be feasting in this abundance of prey, and their numbers will grow disproportionately.
Now the rabbits are under-fed and over-hunted. If you’re unlucky, your hopeful shipments of cuddly creatures might result in all the rabbits on the island dying out. Then, of course, the foxes will starve, and before you know it you’ll have a deserted island.
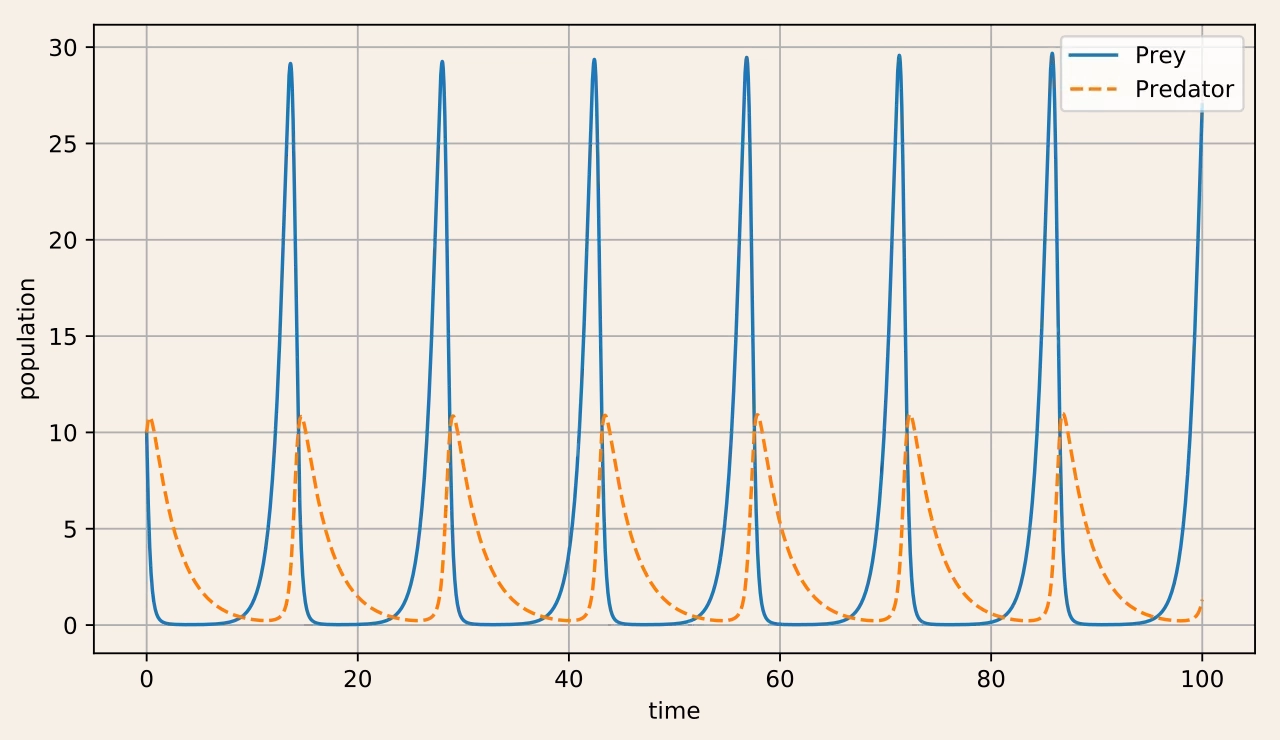
That’s nonlinearity. We’re still far from understanding this kind of predator-prey system completely, despite the fact that it is very simplified, and despite the fact that we’ve studied it to death. Most other real-life emergent systems are even more of a nightmare to assess.
The third reason emergence is difficult is an extension of the nonlinearity problem: chaos. Many nonlinear systems can become chaotic (remember the three-body problem example), which means that tiny initial differences will snowball into very large differences later on. Put differently, in order to predict the system’s outcomes accurately you would need to know all its details with excruciating precision. Make a hair-thin error in your knowledge, and soon that error will make your prediction useless.
This is true if you have exact equations describing the system, and even more true if you don’t. Needless to say, in reality we neither have exact equations nor exact measurements of our systems.
Chaos is the ultimate barrier to our understanding of all things emergent. Even if we could try all possible combinations of systems, and even if we could work out precise equations that explain their nonlinearity, we have little hope to know the systems precisely enough to predict their chaotic behaviors. And sometimes, like the eye of a cyclone, the most interesting emergent behaviors happen right in the midst of chaos.
Reality Emerges
So emergence can be fiendishly difficult to calculate. Even then, I think we shouldn’t treat it as a dark art, a riddle of the ages. We can admire, paint and climb a mountain even when we’re unable to reach its summit or map it accurately; and we can understand emergence and benefit from it even with our weak predictive powers.
Knowing about emergence—seeing the patterns around you as both byproducts of their parts and “things” on their own right—is not only useful in understanding the world, but it also unlocks some fascinating thoughts. Here’s perhaps my favorite:
Reality is emergence, and emergence is reality.
If very physical things around us are emergent, then we need to be careful of what we call “real” and “not real”. If emergence is behind the feel and shape of the materials we touch, the wetness of water, the stability of atoms, the synchronized movement of groups of objects, the evolution of life, the collective behaviors of ecosystems, cities, and factions, then anything else that emerges is equally real. It has an effect on the world, regardless of what its “context” is.
Text on a computer monitor is as physical as text on paper: they have very different contexts (electrons inside grids of pixels vs cellulose fibers and ink) but what emerges in those contexts has the same effect for the reader’s purpose. Online chess is as real as physical chess for the same reason. A simulation of something—say, a VR roller coaster ride—is different but not any “less real” than the thing being simulated. The map of a country is the country, in the constrained sense that you can say “I am here, and I’m going towards this place”. A fictional story is as real as a true story for the purpose of inciting certain emotional reactions in the reader.
The triangle does exist.
An illusion is a physical object.
A hole isn’t the missing of something.
Imagined isn’t the opposite of real.
Eagleman’s story is about a heaven for emergent beings. People and minds are emergent, and so are battalions, teams, and shops. The latter are more than groups of people. They are qualitatively different from the elements that form them, with their own independent reality, laws, and identities. They exists in their own unique contexts, and they “have as little understanding of you as you have of them; they generally have no idea you existed”. â–
📬 Subscribe to the Plankton Valhalla newsletter
Notes
- Cover image: The Dents Blanches At Champéry In The Morning Sun (1916), Ferdinand Hodler.
- All the “tree diagrams” on this page are made with SankeyMATIC.
- In example 6 I mentioned that protons do things very different from wthat individual quarks do. It’s actually very difficult to separate a quark from inside a particle. They are like emergence-making machines. Here is a nice explanation of that.
- If you’re puzzled by my statement that the mass of a proton is not the sum of the mass of its three quarks, this video may clear things up (or puzzle you even more).
- Read this if you’re curious about the physics of human waves.